We seek to elucidate the molecular and cellular mechanisms underlying neuronal communication. We investigate the molecular machines that drive synapse formation, function and plasticity by combining genetic toolkits with electrophysiology and live imaging of neuronal activity. We leverage the power and versatility of the fruitfly Drosophila melanogaster to study glutamatergic synapses at the level of single active zones in identifiable neuronal populations.
Click the video for a quick tour of our lab and to learn more about our research. You can read more in depth about our research here.
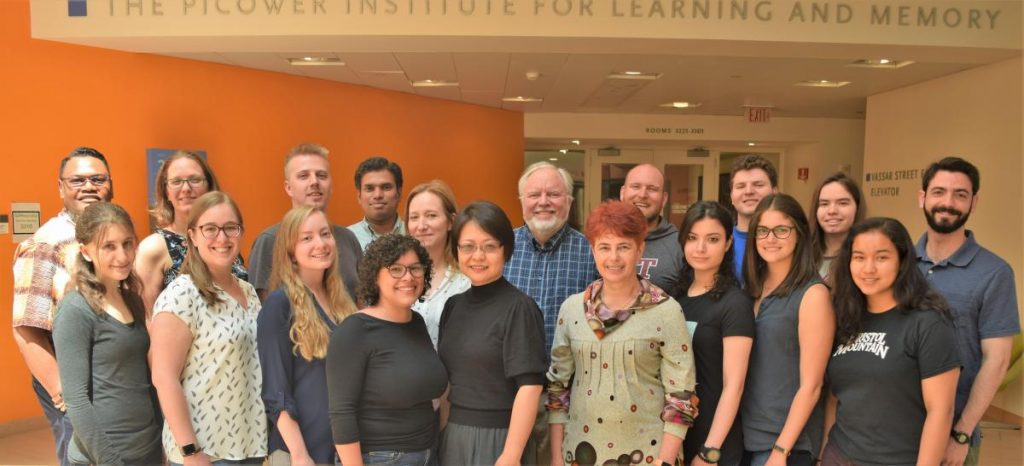
Research Questions
Synaptic Transmission
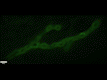
- How does vesicular trafficking control synaptic transmission and neuronal communication? How does calcium influx during an action potential initiate and regulate synaptic communication.
- How do plasticity mechanisms interface with the core molecular machinery for synaptic vesicle trafficking and fusion to alter neuronal communication?
- How does the active zone proteome contribute to and differentially regulate spontaneous and evoked synaptic vesicle fusion?
- How can individual active zones formed by the same neuron show differences in output strength that vary over a 50-fold range?
Synapse Formation
- How do release sites form between neurons and their partners? What molecular and cellular processes regulate active zone number, active zone protein content and active zone release mode?
- How does cell-wide availability of key proteins to growing active zones over the entire neuron interface with capture and retention mechanisms for new material at individual active zones?
- What mechanisms regulate calcium channel trafficking and abundance at single release sites?
- How do these mechanisms differ across distinct neuronal subpopulations with unique synaptic structure, output strength and plasticity?
Disease-relevant research
Glial-neuronal signaling in Epilepsy and Neuronal Excitability
To identify neuronal dysfunctions that cause epilepsy, we have conducted behavioral screens for temperature-sensitive (TS) mutations induced by EMS that result in seizures. We have identified and characterized a glial-specific gene that is required for microdomain glial Ca2+ oscillations. Acute disruption of this protein (termed Zydeco – a NCKX transporter), or acute induction of Ca2+ influx into glia via conditional TRP channels, induces the immediate onset of neuronal seizure activity. Current work indicates glial calcium signaling is likely to interface with the well-known role of glia in regulating ionic balance and neurotransmitter uptake in distinct glial populations.
Neurological Disorders
We have generated Drosophila Huntington’s Disease (HD) transgenic models expressing fluorescently tagged wildtype and pathogenic Huntintin (Htt) proteins that allow for in vivo imaging of Htt localization, axonal transport and aggregate formation in live animals. We identified several pathologies associated with Htt polyQ expression in fly neurons, and have identified small molecules and genetic interactors that can reduce HD pathology in this system. We are also studying mutants of the Drosophila Htt homolog to define the normal function of the protein within neurons.
Autism Spectrum Disorder
Current evidence suggests many loci that predispose to autism are likely to control synapse formation and synaptic plasticity during development. We are taking advantage of genetic manipulations available in Drosophila to explore the mechanisms by which autism-associated synaptic proteins alter neuronal activity or synaptic connectivity. We will use these models to explore how basic synaptic properties may be disrupted in autism-associated genes implicated from human genetic studies.
Lab Life
Between reading papers and performing experiments, lab members enjoy an active social scene in the lab and across MIT. See more here.